*See our 2023 clinical trials review here.
This year marks the 10th anniversary of the development of CRISPR as a genome-editing tool, an achievement that earned Jennifer Doudna and Emmanuelle Charpentier the 2020 Nobel Prize in Chemistry. In the world of developing new medical therapies, 10 years is not a long time, but CRISPR-based therapies have made significant strides. In the first five years, the field focused on refining how CRISPR works in different cell types, improving its efficiency at cutting DNA, and developing CRISPR towards clinical applications for the first time. In the following five years, the discovery and engineering of new CRISPR proteins with different capabilities expanded the CRISPR toolbox and the first CRISPR clinical trials began, yielding sometimes stunning results.
The IGI has been closely tracking the development of new CRISPR-based therapies and the progress of the growing number of clinical trials. Over the past year, trials have started in new disease areas including diabetes and HIV/AIDS. In our annual deep dive into CRISPR clinical trials, we guide you through the current landscape of clinical trials, outcomes, what we hope to learn from each trial, and what is coming next in the world of therapeutic genome editing.
CLINICAL TRIAL BASICS
In the United States, the Food and Drug Administration (FDA) assesses new disease treatments for safety and efficacy through clinical trials on patient volunteers. Early trials (phase 1) evaluate safety and treatment side effects. Later trials (phase 2 and 3) evaluate how effective treatments are and compare new therapies to standard treatments. Sometimes trial phases are combined to expedite testing a treatment.
While the number of CRISPR clinical trials is growing each year, most of the current trials using CRISPR-based treatments are still in early stages. That means that even if the treatments are safe and effective, they’re likely still a few years away from a possible FDA approval and being available to patients in the US.
Learn more about the clinical trial process for genome-editing therapies and the work to expedite the development pipeline in When Will CRISPR Cures Be Available? |
The development of CRISPR genome editing opens up new possibilities in precision medicine. Current trials are underway in seven treatment areas: blood disorders, cancers, inherited eye disease, diabetes, infectious disease, inflammatory disease, and protein-folding disorders.
Before we dive into each treatment area, keep in mind that all current CRISPR clinical trials target specific cells or tissues in individuals without affecting sperm or eggs — that is, no DNA changes are intended to be passed onto future generations.
BLOOD DISORDERS
DISEASE BACKGROUNDS
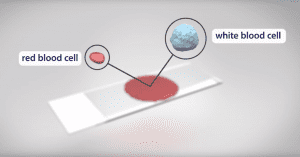
Red blood cells use the protein hemoglobin to carry oxygen from the air we breathe to the rest of the body. Variations in a gene that encodes part of the hemoglobin molecule cause two genetic disorders: sickle cell disease (SCD) and beta thalassemia.
In sickle cell disease, red blood cells are misshapen. Their “sickle” or crescent shape blocks blood vessels, slowing or stopping blood flow. This causes sudden, severe pain crises. Complications of SCD include chronic pain, strokes, organ damage, and anemia. In beta thalassemia, patients do not make enough of the hemoglobin protein, leading to fatigue and anemia. In severe cases, patients suffer organ damage, especially to the bones, heart, and liver. SCD and beta thalassemia can both be fatal.
SCD disproportionately affects certain populations. Globally, the highest level of incidence is in sub-Saharan Africa. In the United States, SCD mainly affects Black Americans. While SCD was the first identified genetic disease, it has received poor research funding and individuals with SCD tend to receive poorer care compared to individuals with other genetic diseases, like cystic fibrosis, that are more likely to affect wealthier, White individuals. New research into genomic treatments for SCD are an important step towards equity.
There are some treatments available for SCD and beta thalassemia, but patients often have severe symptoms and complications even with treatment. Patients with more severe cases of either condition need frequent blood transfusions. Bone marrow transplant can be curative; however, this can only be done when a healthy, matching donor can be found. Bone marrow transplant is not an option for most SCD or beta thalassemia patients.
TREATMENT STRATEGIES
The approach taken to treat these blood disorders with CRISPR technology in the most advanced trial doesn’t directly correct the gene variants that cause disease. It uses a clever workaround: instead of restoring healthy adult hemoglobin, the goal is to increase levels of fetal hemoglobin. This is a form of hemoglobin that fetuses make in the womb, but children and adults don’t make. We don’t know yet why humans switch from one form of hemoglobin to the other after birth, but fetal hemoglobin is not affected by the sickle cell mutation and can take the place of defective adult hemoglobin in red blood cells. This treatment can be used for both SCD and beta thalassemia.
In individuals with SCD, symptoms start to show during infancy, after fetal hemoglobin (HbF) levels decrease. The first step of treatment is to harvest a patient’s blood stem cells directly from their blood. Next, scientists edit the genomes of these cells to turn the fetal hemoglobin gene on. Then, chemotherapy eliminates the disease-causing blood stem cells from the patient’s body. Finally, billions of genome-edited stem cells are put back into their bloodstream. These genome-edited blood stem cells are administered by IV. If it works as intended, these cells will take up residence in the bone marrow, creating a new blood stem cell population which will make edited red blood cells that produce fetal hemoglobin.
This treatment approach is called ex vivo genome editing, because the editing occurs outside of the patient’s body. The advantage of ex vivo editing is ensuring that genome-editing tools only come in contact with the right target cells. It also avoids the risk of long-term presence of CRISPR components in the body, like unwanted edits or immune reactions.
Other trials that are just beginning or will begin soon also use an ex vivo approach, but are different on a molecular level. One uses base editing rather than conventional CRISPR, to turn on fetal hemoglobin. Two other trials aim to directly correct the mutation that causes sickle cell disease using CRISPR Cas-9, restoring healthy, adult hemoglobin.
CURRENT CRISPR CLINICAL TRIALS
In the first use of an ex vivo CRISPR-based therapy to treat a genetic disease, researchers treated an individual with beta thalassemia in Germany in 2019. CRISPR Therapeutics and Vertex Pharmaceuticals are running this trial in Europe and Canada. According to company press releases, at least 14 more individuals have since been treated and followed for at least three months, with five followed for over a year. The first individual with SCD was treated with the same therapy in Nashville, Tennessee in 2019. At least six more individuals with SCD have been treated since then and followed for at least three months, with two followed for over a year.
So far, patient volunteers with both conditions have made remarkable recoveries.
- Patients treated for SCD or beta thalassemia show normal to near-normal hemoglobin levels, where at least 30% (SCD) or 40% (beta thalassemia) of hemoglobin is fetal hemoglobin.
- Patients with beta thalassemia are free from needing blood transfusions. Patients with SCD are free from transfusions and disabling pain crises.
- Molecular tests on bone marrow from each of six patients a year or more after treatment show the continued presence of genome-edited cells
- One patient with beta thalassemia experienced serious immune reactions to treatment which have since resolved. No other serious adverse events were observed, and side effects seem to be related to chemotherapy, not the genome-editing treatment.
- Hear directly from Victoria Gray or Jimi Olaghere, who were both treated for sickle cell disease.
CRISPR Therapeutics and Vertex Pharmaceuticals are jointly running these combined phase 1, 2, and 3 trials in the US, Canada, and Europe. In Europe and the US, this treatment has been given special status to fast-track approval.
Read more:
- CRISPR-Cas9 Gene Editing for Sickle Cell Disease and β-Thalassemia — Frangoul et al., New England Journal of Medicine
- Vertex and CRISPR Therapeutics Present New Data in 22 Patients With Greater Than 3 Months Follow-Up Post-Treatment With Investigational CRISPR/Cas9 Gene-Editing Therapy, CTX001™ at European Hematology Association Annual Meeting — Press release from CRISPR Therapeutics
Three more trials for sickle cell disease will begin shortly. Beam Therapeutics has regulatory approval to move forward with another SCD trial aimed at increasing fetal hemoglobin. Beam uses base editing, a form of CRISPR editing that works without double-stranded DNA breaks and which therefore may be safer than conventional CRISPR.
The other two trials — one from Graphite Bio, and the other from a consortium of researchers from IGI, UCSF, UCLA, and UC Berkeley — will test an alternate approach that would use CRISPR to directly repair the mutation that causes SCD, reverting it to the healthy version. Graphite has begun enrolling patients, and the UC trial will begin recruiting patients later in 2022. The UC consortium trial is the only not-for-profit trial for sickle cell disease.
WHAT TO WATCH FOR
Over the last few years, there has been a burst of research into treatments for blood disorders. Pharma, biotech companies, and academic research institutions are working on conventional and genomic therapies. It’s too soon to say which approaches will be the safest and most effective, but patients are sure to benefit from the renewed research in these disease areas.
The initial results from Victoria Gray, Jimi Olaghere, and other patient volunteers are what genome-editors dream of. If trial data continue to be so positive, the treatment could be approved as soon as 2023.
“Bottom line, the progress of CRISPR/Vertex is a landmark in that it’s likely to generate the first approved CRISPR-based medicine,” says Fyodor Urnov, Ph.D., IGI’s Director of Technology and Translation and a 20-year veteran of the sickle cell field. “That would be an extraordinary moment for us. And it will create a wide road for others in this space — like Beam and the UC Consortium — to rapidly follow suit.”
Long-term follow-up of these and other trial participants is crucial: they will be tracked for years to come to see if the treatment remains effective, and to look for potential long-term side effects which wouldn’t be apparent until further down the line, like cancer from unwanted changes to the DNA. It will be especially interesting to see, for instance, if there are differences in outcomes between patients who receive cells edited with conventional CRISPR versus base editing.
“The main side effects so far have been from the chemotherapy necessary to wipe out the pre-existing bone marrow cells in order for the edited cells to engraft. The chemotherapy is a huge limiting factor for these therapies,” explains Megan Hochstrasser, Ph.D., a CRISPR expert who studied with Jennifer Doudna. “If you have to be in the hospital for weeks because you are getting your bone marrow ablated with chemotherapy, which cripples your immune system, it’s risky, expensive, and time-consuming. That’s a huge barrier to scaling this and making it available widely. It’s a big hurdle that could be overcome if someone finds a way to deliver the treatment directly, without bone marrow ablation.”
Scalability — making enough of a treatment to get it to the many people who need it — will be a major challenge for CRISPR-based treatments for blood disorders, both because of the technical challenges of creating the individualized product and administering the treatment protocol, and the cost. The cost of the current CRISPR-based therapy is in the $1–2 million range, and can only be performed at a small number of medical facilities worldwide, putting it well out of reach of the vast majority of people with SCD or beta thalassemia. Research into in vivo approaches, which could eliminate the need for chemotherapy and decrease the associated risks and expenses, is in early stages, but will be a focus of researchers working to make more widely accessible CRISPR-based therapies for blood disorders in the coming years.
CANCERS
DISEASE BACKGROUND
Cancer refers to diseases that are caused by uncontrolled cell growth. Right now, CRISPR-based therapies are mainly aimed at treating blood cancers like leukemia and lymphoma.
TREATMENT STRATEGY
T cells are a type of white blood cell that have a central role in immune system response. T cells are covered in receptors that recognize other cells as safe or threatening. They patrol the body, killing foreign or dangerous cells, or recruiting other cells to assist. In CAR-T immunotherapy, researchers genetically engineer an individual’s T cells to have a receptor that recognizes their cancer cells, telling the T cells to attack.
The immune system is highly regulated to avoid attacking healthy cells. Some T cell receptors work as “checkpoints” that determine whether an immune response occurs. When a T cell PD-1 receptor comes in contact with a molecule called PD-L1 on another cell, it communicates that it is a “safe” cell and the T cell leaves it alone.
Cancer cells often cloak themselves in these safety signals, tricking the patrolling T cells into ignoring them. Researchers are using CRISPR to edit the PD-1 gene in T cells to stop them from making functional PD-1 receptors so they can’t be tricked by cancer cells. This immunotherapy approach is known as checkpoint inhibition, and it is often used in conjunction with CAR-T engineering to give T cells the greatest possible chance of eliminating cancer.
For these treatments, researchers harvest T cells from a patient’s blood and engineer them in a lab. Then, they put them back into the patient’s bloodstream by IV. Because this treatment relies on ex vivo editing, it is easy to deliver the genome-editing tools to the target cells. CAR-T therapy was approved for use in treating blood cancers in 2017.
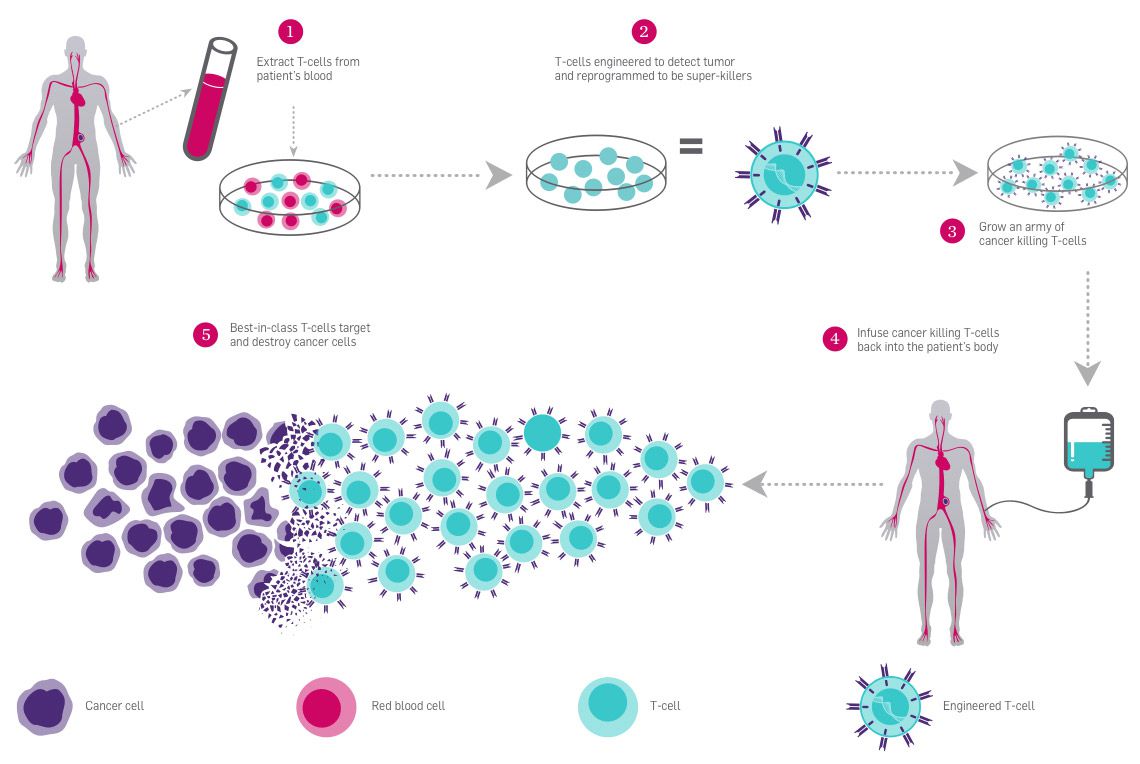
Learn more about CAR-T:
- CAR T-cell Therapy and Its Side Effects — The American Cancer Society
- CAR T Cells: Engineering Patients’ Immune Cells to Treat Their Cancers — National Cancer Institute
CURRENT CRISPR CLINICAL TRIALS
In 2016, an individual with lung cancer became the first person in the world to be treated with a CRISPR-based therapy: this patient was injected with PD-1 edited T cells in a Chinese clinical trial. This and an American clinical trial using CRISPR-based immunotherapies for cancer have been completed. Several other clinical trials using CRISPR-based immunotherapies, mainly to treat blood cancers, are ongoing.
In the Chinese trial, researchers at Sichuan University treated 12 patients with non-small-cell lung cancer with PD-1 edited T cells. This approach did not include CAR-T, as it is not currently an option for lung cancers. Like early stage trials in the US, the main goal was to assess safety and side effects rather than efficacy.
In April 2020, the researchers reported that the treatment was safe to administer and had minor side effects like fever, rash, and fatigue. The intended edit was found with a low efficiency: a median of 6% of T cells/patient before infusion back into the patient. Off-target effects — unintended changes at various places in the genome — also occurred at a low frequency and were mostly in parts of the genome that don’t code for proteins. On-target effects — unintended changes at the target site — were more common (median of 1.69%). 11 out of 12 patient volunteers had edited T cells two months after the infusion, although at low levels. Patients with higher levels of edited cells had less disease progression.
Read more:
- Safety and feasibility of CRISPR-edited T cells in patients with refractory non-small-cell lung cancer — Yu et al., Nature Medicine
- First Trial of CRISPR-Edited T cells in Lung Cancer — Lacey & Fraietta, Trends in Molecular Medicine
The first CRISPR-based therapy trial in the US combined CAR-T and PD-1 immunotherapy approaches, using CRISPR to edit three genes in total. This phase 1 study, run by the University of Pennsylvania in collaboration with the Parker Institute, was completed in 2020. Like the Chinese trial, the goals were to determine if the treatment was safe and had acceptable side-effects, not to cure patients. Two patient volunteers with advanced white blood cell cancer (myeloma) and one with metastatic bone cancer (sarcoma) were treated. Researchers reported that the treatment was safe to administer and had acceptable side effects. The edited T cells took up residency in the bone marrow and remained at stable levels for the nine months of the study. Biopsies on the patient with bone cancer showed that T cells were able to find and infiltrate tumors. Off-target effects were rarely observed. However, unintended edits at the target site were observed frequently, with 70% of cells showing at least one mutation at or near the target site during the T cell manufacturing process. After infusion and over time in patients, the percentage of cells with mutations decreased.
“A really interesting thing is that the American study did show a percentage of the large genomic rearrangements that people fear,” says Hochstrasser. “But the percentage of cells with these changes actually decreased over time. It seemed like the cells that had those types of mutations were dying or getting out-competed by the other cells. So, it seemed like the cells that you wouldn’t want in the body were not actually sticking around in the body, which was a surprise to me, and very encouraging.”
Read more:
- CRISPR-engineered T cells in patients with refractory cancer — Stadtmauer et al., Science
The therapies in the two trials described above are autologous: cells are taken from each patient, edited, multiplied, and then put back into the same patient. This process is expensive, time-consuming, and few facilities can do it. Sometimes the manufacturing process — which is starting with cells from a sick patient — just doesn’t work, produces low potency cells, or individuals die of their disease while waiting for the manufacturing process to be completed.
In October 2021, CRISPR Therapeutics announced results from their ongoing US-based Phase 1 trial for an allogeneic T cell therapy. Allogeneic therapies are made from cells from a healthy donor. These cells are edited to attack cancer cells and avoid being seen as a threat by the recipient’s immune system, and then multiplied into huge batches which can be given to large numbers of recipients. Allogeneic products reduce cost, time until treatment, and potentially provide more consistently potent cells. Allogeneic therapies are sometimes referred to as “off-the-shelf.”
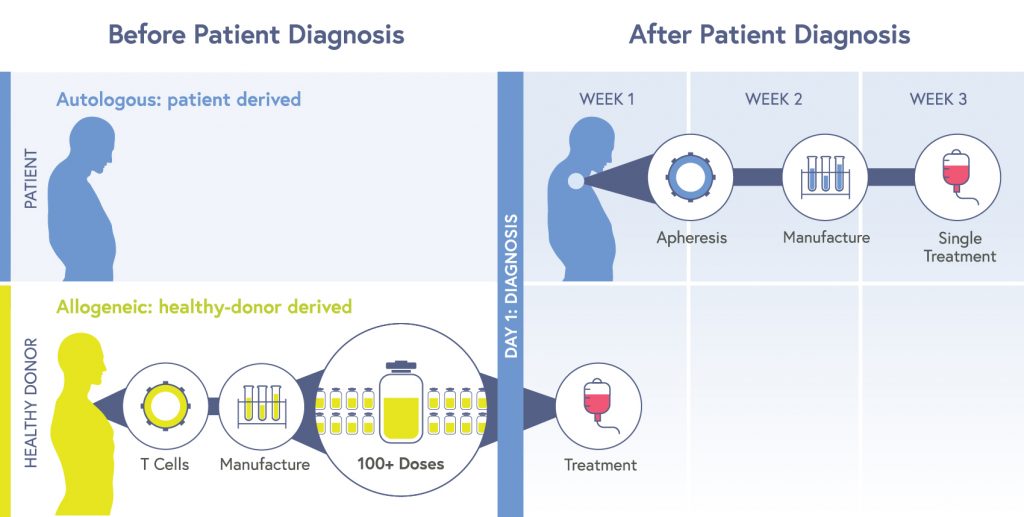
The press release from CRISPR Therapeutics gave preliminary results for individuals with lymphomas who had been treated and followed for at least four weeks after treatment. Side effects were not severe, and the safety profile was superior to other CAR-T products. In these patients, almost 60% showed a positive response to treatment, with 21% showing no sign of disease for six months after a single treatment. This is similar to approved autologous CAR-T therapies made without CRISPR technology.
Together, these studies indicate that CRISPR-engineered CAR-T therapy may be a promising line of treatment: they appear to be fairly safe, the side effects are tolerable, and the treatment does not tend to induce a strong immune reaction.
WHAT TO WATCH FOR
The FDA has already approved CAR-T therapies and PD-1 pathway inhibitors that don’t use genome editing. In other words, the proof-of-principle work for these therapies has already been done successfully.
The efficiency of editing — meaning, the percentage of cells that actually got edits — was poor in both autologous trials. But these trials were done using technology from 2016, and there have been significant improvements over the last six years. These trials are an important proof of concept about the immediate safety and tolerability of the treatment, but hopefully new trials will show improved editing efficiency.
If researchers get better editing efficiency, will genetic checkpoint inhibition work as well or better than checkpoint-blocking drugs? Will PD-1 editing be as or more effective than antibody treatments that disable PD-1? Future research will have to answer these questions. And while right now CRISPR-based CAR-T does not provide an advantage over conventional CAR-T, CRISPR provides options to develop T cell therapies in ways that are not possible with conventional gene therapy. Researchers are working on CRISPR-editing T cell therapies where genes are added at specific locations in the genome, or using base editing to make changes to multiple genes at once.
The push towards allogeneic, or off-the-shelf, treatments is particularly interesting, given the possibility for quicker and broader access. We will be sure to keep an eye on the development of this and other allogeneic cancer immunotherapy products.
There are two more big areas where CRISPR-based immunotherapies for cancer are heading. The first is targeting solid tumors, with at least three early stage trials going on currently. Solid tumors are a tougher challenge than blood cancers. First, in blood cancers, the cancerous cells are easier for immune cells to reach. In solid tumors, immune cells have to infiltrate a solid mass that isn’t friendly to T cells. Second, scientists are still trying to find ways to send T cells specifically to solid tumors. And finally, when T cell therapy is effective, it kills cancer cells. When a high number of cells are killed at once — from a big tumor, or multiple smaller tumors — the dead cells can cause a dangerous inflammation reaction. We’ll definitely be keeping an eye out for safety and side effect data from the new trials.
“People have been thinking of various ways to boost T cell functionality,” says Alex Marson, M.D., Ph.D., IGI’s Director of Human Health and a Professor of Medicine at UCSF. “CRISPR may be useful for adding or removing genes to make T cells more powerful in solid tumors. We’re looking for ways to enhance the functionality and persistence of T cells, and the safety of this approach.”
The other development to keep an eye out for is moving immunotherapy beyond T cells. “Another thing coming down the road is using different cell types,” says Marson. In addition to allogeneic products from healthy donors, researchers are working on developing natural killer cells and stem cell-derived cells to target cancers.
GENETIC BLINDNESS
DISEASE BACKGROUND
Leber Congenital Amaurosis (LCA) is the most common cause of inherited childhood blindness, and LCA10 is the most common form of LCA. This disease is caused by a single nucleotide mutation in a photoreceptor gene, leading to serious vision loss or blindness within the first few months of life.
Photoreceptor cells in the eye convert light into nerve signals that travel to the brain. In LCA10, the photoreceptor gene has a mutation, leading the cells to make a shortened, faulty version of a crucial protein. This faulty protein makes the photoreceptor cells dysfunctional. When patients with LCA10 receive light to the eye, the dysfunctional photoreceptor cells can’t send all of the necessary messages to the brain. Without good communication between the eyes and the brain, patients experience vision loss or blindness.
TREATMENT STRATEGY
The CRISPR treatment for LCA10 makes a change to the patient’s defective photoreceptor gene so that it makes a full-size, functional protein instead of the short, broken version of the protein. If enough cells are edited to make the healthy protein, the hope is that patients will regain vision.
Patient volunteers receive a single dose of the CRISPR therapy by injection directly into the eye. The injection contains a nonpathogenic virus (AAV) carrying the Cas9 protein and its guide RNA. Viruses are often used in gene therapy and genome editing because they have a natural ability to get into cells. For the LCA10 treatment, the viral vector is engineered so the therapy is active only in photoreceptor cells.

This approach is an in vivo treatment, meaning the genome editing occurs inside the patient’s body. Compared to ex vivo editing, in vivo editing has more challenges and different risks. One of the biggest risks is that viral delivery tools or genome editing components will provoke dangerous immune reactions in a patient. Another big challenge is finding ways to stop the CRISPR enzymes from sticking around for too long, since that would give them a greater chance of making unwanted cuts in the DNA.
Though needles near eyeballs makes most people a little queasy, the eye is actually an ideal organ for in vivo editing. It is small, so it only requires a single-dose, small-volume treatment. The eye has less immune reactivity than most tissues, making a dangerous immune reaction less likely. And because the eye is relatively contained, the CRISPR components aren’t likely to travel to other parts of the body, so there is a lower risk of unwanted genome editing or immune responses in other tissues. In experiments on a mouse model of LCA with the same mutation, researchers found that ~10% of cells showed the desired edit — this is thought to be the minimal percent needed to get some vision restoration. The treatment showed few side-effects in animal models, and studies in human retinal cells showed no off-target effects at over 100 sites with similar sequences. See preclinical data here.
CURRENT CRISPR CLINICAL TRIAL
This is the first in vivo CRISPR therapy trial, meaning, the first time CRISPR is being used to edit someone’s genes within their own body. The first patient volunteer in this US-based study, sponsored by Editas Medicine, was given a low-dose of the treatment in March 2020. Dosing of two patient volunteers in the first, low-dose cohort was completed by November 2020 and dosing of four patient volunteers in an adult mid-dose cohort followed, completed by June 2021. Starting with a low dose reduces the risk of dangerous side effects throughout the trial. Enrollment for a high-dose adult cohort (four patient volunteers) and a pediatric cohort (four patients) began in June 2021, after safety was established from dosing adult patients with low and mid doses. Dosing of the new cohorts is expected to be completed by July 2022. Patients are dosed in a single eye, with the other eye serving as a control to test the vision of the treated eye against.
No papers have been published sharing trial data, but Editas has issued press releases and presented data at two conferences in fall 2021. According to press release materials, no serious adverse events or dose-limiting toxicities had been observed.
Efficacy is challenging to evaluate in these individuals. Because their vision is so reduced, the classic line-by-line letter reading eye test you may be familiar with cannot be used. A number of other tests, including mobility (e.g., ability to navigate around objects in one’s path) and ability to detect light were used. Researchers are still honing in on the best way to assess vision change in these patients. In two of the three mid-dose subjects who were followed for at least three months, there were improvements in some of the vision assessments. Hear directly from trial participants Carlene Knight and Michael Kalberer here.
Learn more:
- BRILLIANCE: A Phase 1/2 Single Ascending Dose Study of EDIT-101, an in vivo CRISPR Gene Editing Therapy in CEP290-Related Retinal Degeneration — Data presentation from Editas
WHAT TO WATCH FOR
The FDA is satisfied with the initial safety data, but long-term safety is unknown. Because this treatment is delivered with a viral vector, there will be ongoing expression of CRISPR-Cas components in the eye. Ongoing expression presents a higher risk of unwanted DNA edits, and, perhaps more crucially, of immune reactions to the viral vector and/or the Cas protein over the long-term. It will be necessary to follow these patient volunteers for years to come to see how they fare.
In terms of efficiency and off-target effects, there is currently no way to directly assess what percentage of cells are being edited or whether there are unwanted edits in living patient volunteers. Inferences about the efficiency of editing can only be made relative to how much vision improvement there is among patient volunteers. Researchers are following individuals who have already been dosed to track whether improvements in vision are stable over time, improve, or are lost.
This trial came on the heels of the FDA approval of Luxturna, a gene therapy product for a different inherited retinal disease that causes vision loss. “The retina is a promising place for molecular therapies. In fact gene augmentation [for Luxturna patients] had such dramatic improvement that the FDA rapidly approved it,” says Bruce Conklin, M.D., IGI Deputy Director and Gladstone Institutes Investigator. “But LCA10 was a much tougher target, since the vision loss is so great that the brain actually loses its functional writing to the eye. So, even if the therapy is successful in the retina, the brain can not process the information.” In other words, because the extent of vision loss is more severe in LCA10 patients, and because vision loss occurs earlier in development, it is likely that the part of the brain that processes visual information has not developed all the necessary connections for vision. So, even if the retina issue is corrected, the brain of individuals with LCA10 may not have the ability to process much visual information.
The company reported their data of improvement on some measures in two patients in an optimistic light. Hear from those individuals here. That said, the reception by the scientific community was mixed. Some saw the results as a step in the right direction; others saw it as a deep disappointment. Hopefully the next steps of the trial — treating individuals with LCA10 at a younger age (pediatric cohort) and/or with higher doses (adult high-dose cohort) — will yield more dramatic and definitive improvements. Higher dose treatments may prove more effective, although they are also more likely to provoke stronger immune reactions against the treatment. In all scenarios, the surgery necessary to administer the therapy is complex and may prove to be the major risk of this approach.
DIABETES
DISEASE BACKGROUND
When we eat, nutrients from our food enter our bloodstream. Insulin is the crucial molecule that ferries sugar from the bloodstream into our cells for use as energy. Beta cells in the pancreas make insulin.
Type 1 diabetes (T1D) is an endocrine disorder that occurs when pancreatic beta cells are destroyed, usually by an autoimmune reaction. Without enough beta cells, the body cannot make enough insulin. Individuals with T1D must carefully monitor blood sugar and insulin levels for their entire lives, avoiding life-threatening blood sugar highs and lows by carefully timing meals, exercise, and self-dosing of insulin. T1D is not caused by diet, exercise, or weight, and cannot be controlled through lifestyle changes alone. Kidney damage, nerve pain, damage to blood vessels and the heart, vision loss, and limb amputation are common complications of T1D.
TREATMENT STRATEGY
Researchers have long been interested in transplanting healthy pancreatic cells into individuals with T1D. While ongoing clinical trials in this area show that pancreatic cell transplantation can greatly benefit individuals with T1D, individuals who receive conventional pancreatic cell transplants must take drugs that suppress the immune system on an ongoing basis so that their body does not attack the transplanted cells. Immunosuppressant drugs can have serious side effects, including increased risk of dangerous infections and cancers.
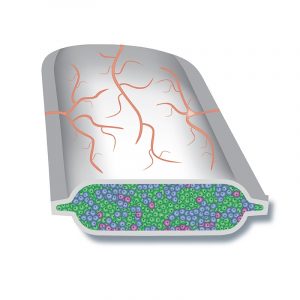
In the new CRISPR treatment strategy, pancreatic cells will be made from stem cells. CRISPR will be used to edit the immune-related genes of these cells so that the patient’s immune system does not attack them. These cells will be implanted into the patient’s body in a special pouch. Blood vessels will grow along the outside of the pouch, bringing the cells oxygen and vital nutrients from the blood, and taking up insulin from the cells. The aim is for patients to have healthy new pancreas cells to help control or even cure their T1D without having to take immunosuppressants.
CURRENT CRISPR CLINICAL TRIAL
There is currently one clinical trial for T1D, sponsored by CRISPR Therapeutics and ViaCyte, Inc. The first patient volunteer was treated in Canada earlier this year. This is a phase 1 trial, which will assess safety, side effects, and whether the cells are able to avoid attack by the immune system. This trial represents the first use of CRISPR to treat an endocrine disease.
WHAT TO WATCH FOR
Conventional pancreatic cell transplantation is already being tested in clinical trials with some strong signs of improvement in T1D patients: some are able to stop self-administering insulin altogether and those who are not still report improvement in managing blood sugar.
There are two main differences in this trial: 1) the transplanted cells are derived from stem cells and 2) CRISPR is used to edit the cells to avoid detection by the patient’s immune system. If this works, patients could have the benefit of transplantation — improvement or even a cure for T1D — without the risks and side effects of immunosuppressants. Early data from ViaCyte’s other trials of implanted stem cells sans CRISPR edits show positive indications that the cells are safe and turn into mature insulin-producing cells. Whether the cells in this trial can successfully evade detection by the immune system will be the most crucial outcome to watch.
If this treatment is successful, it has a scalability advantage over conventional transplants. In conventional transplants, cells come from a deceased donor on an individually-matched basis or the patient’s own cells are harvested and edited, a highly technical process that few facilities can do. In this trial, the pancreatic cells are made from a stem cell source that could be given to any patient in need. In other words, the pouch of edited cells could be an off-the-shelf type product, rather than an individually manufactured product. Off-the-shelf products are quicker, easier, and cheaper to make than personalized products and could increase the accessibility of this therapy.
INFECTIOUS DISEASE – CHRONIC UTI
DISEASE BACKGROUND
Urinary tract infections (UTIs) are a common infection causing over 8 million visits to health care providers every year. UTIs occur when bacteria that shouldn’t be there take up residence in the bladder, kidneys, the tubes that connect the bladders to the kidneys, or the tube through which urine exits the body. E. coli, a common fecal bacteria, is usually the culprit. For anatomical reasons, UTIs are much more common in women.
UTIs cause a burning sensation during urination and the need to urinate frequently. Beyond discomfort, they can become dangerous if they affect the kidneys or if bacteria enter the bloodstream. Most UTIs are easily treated with a short course of antibiotics, but sometimes antibiotics are ineffective or the infection keeps recurring, known as chronic UTI.
TREATMENT STRATEGY
The treatment currently in clinical trials is a cocktail of three bacteriophages combined with CRISPR-Cas3, designed to attack the genome of the three strains of E. coli responsible for about 95% of UTIs. The destruction of the genome kills the bacteria.
Bacteriophages, or phages for short, are viruses that attack bacteria. They usually work by injecting their genetic material into bacteria and using the bacteria as a factory to make more bacteriophages. Eventually, the bacteria will burst, dying as they release more copies of the phage. Phages are being developed for use against bacterial infections, and have gotten more attention recently as antibiotic resistance has become a major public health threat.
In this treatment, phages have been engineered to be an even more powerful tool against E. coli. In addition to the natural action of phages that kills bacteria, these bacteriophages contain CRISPR-Cas3 in their genome. While the more-famous Cas protein Cas9 makes a precise cut at a single location, Cas3 shreds DNA at the gene regions it is targeted to find. In this treatment, the CRISPR-Cas3 system is made to target the genomes of the targeted E. coli strains and damage them by shredding stretches of DNA. In experiments on isolated cells and in animals with urinary tract and other infections, the addition of CRISPR-Cas3 makes phages much more effective at killing E. coli. Locus Biosciences delivered the treatment directly to the bladder by catheter in the phase 1 trial.
CURRENT CRISPR CLINICAL TRIAL
This is the first trial using a CRISPR-based therapy to treat infection. It is also the first trial to use the Cas3 protein, which targets longer stretches of DNA for destruction, rather than Cas9, which makes a precise cut at one location.
Phages have been considered a possible antibacterial therapy since they were first identified about 100 years ago, but the discovery of antibiotics like penicillin, as well as the difficulty of patenting phages, limited the development of phage therapies. Over the past decades, phages have occasionally been used by doctors for what is known as “compassionate treatment.” Compassionate treatment is when an unapproved drug or therapy is used to treat a seriously ill individual when no other treatments exist. At least 25 case reports of compassionate phage therapy have been published in the last 20 years. Some reports claim success at healing patients, but under compassionate treatment, clinicians use different phages in different amounts for different conditions — clinical trials are necessary to really evaluate the safety and efficacy of phage treatments.
As resistance to traditional antibiotics like penicillin becomes an increasing public health threat, there is renewed interest in developing and testing phage therapies. Phages could in some ways even be preferable to effective antibiotics, because each phage usually only kills a specific kind of bacteria. Antibiotics are typically destructive to healthy bacteria as well, whereas phage therapy has the potential to be much more targeted and precise. In addition to innovations using CRISPR technology, this trial is significant because it is one of the first few well-controlled clinical trials for phage therapy, and the first to combine the CRISPR system with phage therapy.
Locus Biosciences completed their US-based Phase 1b trial in February 2021. In press releases, they reported that results of the trial supported the safety and tolerability of the new therapy, with no drug-related adverse effects. No data have been published yet, but Locus says the initial results show a decrease in the level of E. coli in the bladder of patient volunteers given the CRISPR-based treatment. A representative of Locus Biosciences has confirmed that the company is moving forward with a phase 2/3 trial, expected to begin recruiting patients by June 2022. Combining phases 2 and 3 helps shorten the time until potential FDA approval for the therapy.
WHAT TO WATCH FOR
The completed trial was Phase 1, which means it was designed mainly to test whether the treatment is safe and has tolerable side effects, not how effective the treatment is. However, the results from the trial indicate that the therapy can decrease the level of E. coli in the bladders of infected patients. Researchers at Locus also used this trial to do careful analysis of how quickly phages can multiply within the urinary system — this is the kind of information about phage therapies that is needed for the field to advance.
While Cas3 can destroy longer stretches of DNA, Cas9’s ability to make double-stranded breaks could also be effective at killing bacteria, but at the moment there is no comparative data. “Perhaps Cas3 could better prevent any straggling survivors that somehow managed to repair the Cas9 cut,” says Hochstrasser. “But I’d be curious to know if there is a difference, because it’s part of a huge system that could be hard to deliver in other contexts.”
INFECTIOUS DISEASE – HIV/AIDS
DISEASE BACKGROUND
Human immunodeficiency virus, commonly referred to as HIV, is a virus that attacks the body’s immune system. HIV infects CD4 T lymphocytes, a type of immune cell that is important for fighting infections. HIV makes copies of itself inside the CD4 cell and then kills the cell, releasing more copies of the virus to infect and kill other CD4 cells. If HIV is untreated, it can lead to acquired immunodeficiency syndrome (AIDS), a condition where the immune system is severely damaged and an individual can get very sick or die from common infections. Individuals with AIDS are also vulnerable to rare infections and cancers that are not seen in people with healthy immune systems.
AIDS was first recognized in gay men in the early 1980s, and it took years of activism to combat social stigma and get significant research funding. In 1987, the first treatment that inhibits replication of HIV was approved by the FDA. There are now six types of drugs used to treat HIV/AIDS, often in combination. These drugs are remarkably effective at reducing the amount of virus an HIV positive person has, allowing many HIV positive people to live long, healthy lives. However, HIV is a retrovirus, which means it stores copies of its own genetic material within the host’s own genome in an inactive form. Even when individuals have such low levels of the virus that it is undetectable in blood tests, their own cells act as a “reservoir” from which the virus can be reactivated at any time. Because of this, it is currently not possible to eliminate HIV from the body. Antiviral treatments, which can have serious side effects over time, must be taken on an ongoing basis by the ~1 million Americans who are HIV-positive.
TREATMENT APPROACH
In experimental treatments, the approach is to use CRISPR genome-editing molecules to target the HIV DNA sequence stored in the host cell genome. The guide RNAs direct the Cas9 protein to cut at two sites within the HIV genome, surgically excising most of the genome and effectively eliminating HIV from the cell. The CRISPR treatment will be delivered by an AAV9 viral vector and administered as in vivo treatment by infusion.
CURRENT CRISPR CLINICAL TRIAL
This is the first trial targeting a retrovirus, and it is sponsored by Excision Biotherapeutics. It is currently open for enrollment, with an aim of enrolling ~9 patient volunteers at locations across the US. As a phase 1/2 trial, the goals are to evaluate safety and side effects, correct dosage, and efficacy of the treatment at excising the virus out of cells.
WHAT TO WATCH FOR
Since researchers have learned about the HIV “reservoir,” their goal has been to eliminate it to cure patients. In terms of efficacy, we have every reason to think that CRISPR will be able to disrupt and cut the HIV genetic material in its hiding place in the human genome. But the big question is what percentage of cells will it be able to reach? Can it edit enough cells to eliminate the infection? This is a much tougher problem than treating conditions like blood disorders where getting enough cells making enough healthy protein is sufficient for a cure. Editing less than half of blood stem cells seems to be enough to effectively cure sickle cell disease or beta thalassemia. But for the HIV treatment to be curative, a CRISPR therapy needs to eliminate the HIV genetic material from almost every cell where it is hiding. Many in the field are highly skeptical that this approach can work.
One common concern about genome-editing therapies is off-target effects: unwanted edits made by the genome-editing components. This trial is only the second systemic administration of a CRISPR treatment. In most other treatments, specific types of cells are edited outside of the body, and then put back in after editing (like treatments for blood disorders, cancer, or T1D) or genome-editing therapies are delivered to self-contained organs (like treatment for genetic blindness and chronic UTI). Systemic delivery exposes a wide variety of body tissues to genome-editing components, making the risk of off-target effects higher than for non-systemic approaches.
“This is a viral-delivered Cas9 — a highly immunogenic protein — with what, as best as we can tell, is a ubiquitous promoter,” says Urnov. “It’s different than an in vivo editor making cuts in the eye, which is an immunoprivileged, self-contained setting.” In other words, when CRISPR-based treatments are delivered by a virus and with this particular genetic sequence, the genome-editing components can persist in many parts of the body for a long time. The longer they are in the body, and the more widely spread, the greater the opportunity for unwanted edits and immune reactions against the CRISPR components. The individuals in this trial will be followed for years to come to monitor for any long-term health effects that could be associated with unwanted changes to the DNA.
This trial will be done on patients who are responding well to antiretroviral treatments. If this phase goes well, future trials will likely aim to take patients off of their medications and assess if the virus is able to rebound or has been eliminated from the body.
Another area of CRISPR research that may eventually help individuals with HIV or other chronic infections is editing T cells to help control infections. For HIV, this could come in the form of editing different kinds of T cells to better eliminate the virus or to be more resistant to becoming infected.
PROTEIN-FOLDING DISEASE – HEREDITARY TRANSTHYRETIN AMYLOIDOSIS
DISEASE BACKGROUND
Hereditary transthyretin amyloidosis (hATTR) is a fatal disease caused by mutations in a single DNA letter in the gene TTR.
When the TTR gene is mutated, it makes a protein that folds the wrong way. The incorrectly folded proteins stick together, forming clumps called amyloid fibrils, in a process called amyloidosis. The protein clumps accumulate in organs and tissues, where they interfere with normal functions. ATTR has similarities to other neurological diseases involving protein misfolding and amyloidosis including Alzheimer’s and Parkinson’s diseases.
Individuals with ATTR first have symptoms in early or middle adulthood. Symptoms vary, but usually include severe effects on the nervous system and/or heart. Nerve pain, loss of movement control, digestive problems, vision loss, dementia, and heart failure are common in ATTR. Damage to the nervous system and/or heart eventually kills the individual.
ATTR usually occurs spontaneously, but for some individuals, the mutated gene is passed down from their parents. This leads to hereditary ATTR, or hATTR. Often, hereditary forms of neurological diseases are better understood than spontaneous cases because they are easier to study. hATTR is a rare disease, affecting about 50,000 people worldwide.
TREATMENT APPROACH
The treatment being investigated uses CRISPR-Cas9 tools to reduce the amount of faulty TTR protein the body makes. Less faulty TTR means less protein clumps (amyloidosis). The treatment is delivered in a single dose by IV.
The aim of this hATTR treatment isn’t to fix a gene: it’s to break the gene so that patients stop making the faulty protein altogether. The CRISPR components cut the TTR gene, creating a double-stranded break in the DNA. As the cell tries to repair the DNA without a corrected template, the repair attempts mutate the gene even more. And when a gene is too badly damaged, a cell will sometimes stop making the protein it codes for.
Getting the CRISPR components into cells is a big challenge for in vivo genome-editing therapies. Many treatments in development use viruses to deliver the genome-editing components. This is the first clinical trial for a CRISPR-Cas9 therapy delivered in a lipid nanoparticle. The lipids, or fat molecules, surround the gene-editing components, and are able to get into the cell.
In animal models, lipid nanoparticles have a tendency to accumulate in the liver. TTR is primarily made in the liver, so researchers are taking advantage of this to naturally get the treatment to where it is needed in the body.
CURRENT CRISPR CLINICAL TRIAL
This is the first trial that uses lipid nanoparticles to deliver the genome-editing treatment. It is also the first trial to deliver genome-editing components systemically, that is, to the whole body rather than to one specific type of cell or tissue.
The phase 1 trial is sponsored by Intellia Therapeutics in collaboration with Regeneron Pharmaceuticals. The first patient volunteer was dosed in November 2020 in the United Kingdom. In total, data has been shared on fifteen patient volunteers, who received one of four potential dosages of the gene-editing reagents. Most adverse events were mild. All patient volunteers showed a reduction in TTR protein levels, with higher doses leading to greater reductions in the protein. The protein level was reduced by an average of 87% in individuals who received the highest dose. In amyloid disorders like TTR, the level of precursor proteins is related to clinical outcomes. In other words, if the patient volunteers continue to produce less TTR protein, it is very likely that they will have less severe disease. Intellia CEO John Leonard hopes that with dramatic reductions in toxic proteins being produced, people’s bodies will be able to clear out the toxic protein and even reverse damage done by the disease, but it’s too soon to tell. Currently, more patient volunteers are being enrolled in the phase 1 study.
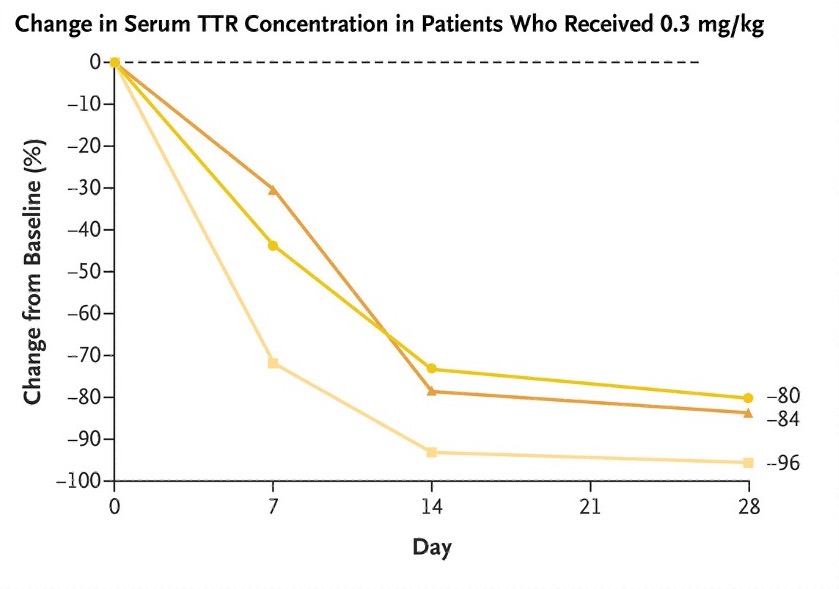
Read more:
- CRISPR-Cas9 In Vivo Gene Editing for Transthyretin Amyloidosis — Gillmore et al., New England Journal of Medicine
WHAT TO WATCH FOR
This is the first experimental CRISPR therapy to be administered systemically to edit genes inside the human body. In other treatments, specific types of cells are edited outside of the body, and then put back in after editing (like treatments for blood disorders and cancer) or genome-editing therapies are delivered to self-contained organs (like treatment for genetic blindness and chronic UTI). These strategies help ensure that only the cells or tissue of interest are edited.
One common concern about genome-editing therapies is unwanted edits made by the genome-editing components. This is particularly a concern with CRISPR treatments delivered by viruses, since the genome-editing components may persist in the cell for a long time, giving them more opportunity to make editing errors. Avoiding systemic delivery helps reduce the overall risk.
In this trial, risks are reduced because 1) lipid nanoparticles tend to aggregate in the liver, which is the tissue being targeted in ATTR treatment and 2) no viruses are used. In animal studies of the hATTR treatment delivered by lipid nanoparticles, the genome-editing components were cleared from the body in less than a week, dramatically reducing the chance of unwanted edits. Another risk of systemic administration of editing reagents is the potential to trigger a dangerous immune reaction: so far, the outlook is sunny as no trial participants have experienced any serious side effects.
Efficiency — meaning, what percentage of cells are edited — is a big question. In nonhuman primates, only 35–40% of liver cells need to be edited to reduce TTR levels enough to have a therapeutic benefit. There are no reports yet on what percentage of cells are edited in these patients, but the strong reductions in TTR protein are extremely encouraging as indicators of efficiency of editing and efficacy of the treatment. We’ll be watching closely to see whether these reductions in TTR protein last over time and lead to changes in real-world outcomes for trial participants.
The early positive indicators from this trial are encouraging for treating other disorders where editing liver tissue may be beneficial. Hear directly from Patrick Doherty, a trial participant.
INFLAMMATORY DISEASE – HEREDITARY ANGIOEDEMA
DISEASE BACKGROUND
In hereditary angioedema (HAE), an individual has severe attacks of inflammation, leading to swelling. Swelling usually happens in the arms and legs, face, intestines, or airway. Swelling of the intestines can lead to severe pain, nausea, and vomiting, and swelling of the airways can be life-threatening. Individuals with HAE usually begin to get attacks in childhood. Without treatment, attacks occur every 1–2 weeks, lasting 3–4 days each. HAE affects about 1 in every 50,000 people.
There are three known types of HAE. Types I and II are caused by mutations in the gene that makes the C1 inhibitor protein. In healthy individuals, proteins that increase and decrease inflammation are in a careful balance, helping the body respond to threats and injuries to just the right degree. The C1 inhibitor protein helps reduce inflammation. In HAE, mutations lead to lower levels of C1 inhibitor protein. Without enough C1 inhibitor protein, the protein bradykinin accumulates in the blood. Bradykinin makes fluid leak from the blood vessels into body tissues. When this happens excessively, it leads to HAE swelling attacks.
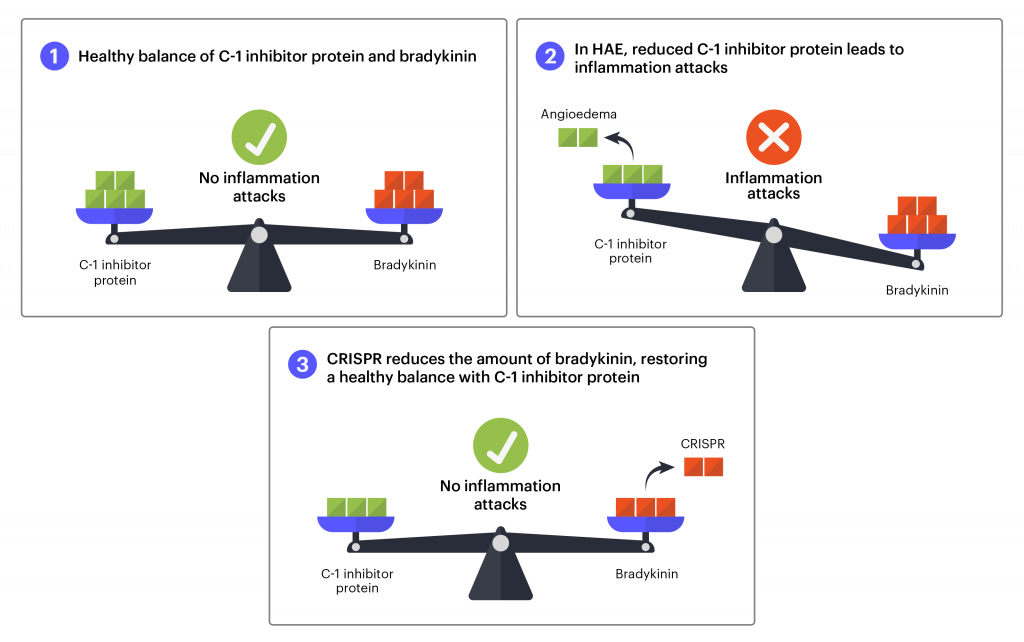
Current treatment options require daily pills or intravenous (IV) or injection administration as often as twice per week. Even when administered regularly, individuals with HAE may still experience occasional attacks.
Like hATTR, angioedema can happen spontaneously, but for some individuals, the mutated gene is passed down from their biological parents. This leads to hereditary angioedema. HAE is a rare disease, affecting about 150,000 people worldwide.
TREATMENT STRATEGY
The treatment that is currently in clinical trials uses CRISPR-Cas9 tools to reduce the amount of bradykinin protein the body makes. Less bradykinin means less inflammation and swelling. The treatment is delivered in a single dose by IV. As in the hATTR treatment, the aim isn’t to fix a gene, but to break a gene to stop the disease process.
The CRISPR components cut the TTR gene, creating a double-stranded break in the DNA. As the cell tries to repair the DNA without a corrected template, the repair attempts mutate the gene even more. And when a gene is too badly damaged, a cell will sometimes stop making the protein it codes for.
The protein prekallikrein is processed into kallikrein, which is crucial to making bradykinin. In this treatment, the aim is to make the gene that codes for prekallikrein nonfunctional (knock-out), reducing the amount of kallikrein, and ultimately reducing the amount of bradykinin. CRISPR-Cas9 is used to make a cut in the prekallikrein gene and when the body tries to repair the DNA break, it renders the gene nonfunctional. If this works as intended, it will reduce the amount of bradykinin, bringing it back into balance with C1 inhibitor protein to prevent inflammation attacks. Previous research shows how much kallikrein needs to be reduced to prevent attacks. In Intellia’s work in nonhuman primate models, a single dose of the CRISPR-based treatment can reduce kallikrein protein to this level, and the reduction was sustained for more than a year.
In this trial, the CRISPR-Cas9 reagents are delivered by lipid nanoparticles. As in hATTR, the aim is to edit cells in the liver. Lipid nanoparticles have a natural tendency to accumulate in the liver, so researchers are taking advantage of this to get the treatment to where it should be. This is an in vivo, systemic treatment, administered intravenously.
CURRENT CRISPR CLINICAL TRIAL
This phase 1/2 study is being sponsored by Intellia Therapeutics, enrolling up to 55 patient volunteers in New Zealand. The first phase will test two different doses of the treatment, looking at safety and side effects. The second phase will compare the efficacy of the treatment relative to placebo.
WHAT TO WATCH FOR
This trial uses the same design as Intellia’s trial for hATTR: CRISPR-Cas components packaged in lipid nanoparticles, administered by IV. The lipid nanoparticles accumulate in the liver, which is where researchers are hoping editing will occur. This is an example of a platform technology: using the same components, delivery, and administration method for a treatment, while just changing onecomponent to adapt the therapy to a different disease. For CRISPR-based treatments, the variable component is typically the guide RNA that determines where a DNA cut will be made. The early success of Intellia’s hATTR treatment at reducing the target protein is a positive indicator for the lipid nanoparticle/IV administration platform for liver editing.
An advantage of both the hATTR trial and this trial (HAE) is that each has a helpful biomarker that can be measured. A biomarker is a molecule in the blood, urine, or other body fluid that can be measured as a diagnostic to assess how well a treatment is working. For HAE, researchers will use blood testing to see if the genome-editing components are successfully reducing the levels of proteins that cause inflammation. This is a real advantage over trials like the LCA10 trial where researchers have no direct way to assess if the successful genome editing is occurring in a living subject. In the HAE study, researchers will also track the number of inflammation attacks after treatment.
THE BIG PICTURE
CRISPR genome editing is only 10 years old, but we are already seeing remarkable progress. Each year, this article gets longer as more trials launch and therapies expand into new disease areas. Taken together, these CRISPR clinical trials are helping scientists learn about the types of DNA changes CRISPR enzymes make in different cells, (including unwanted off-target changes and problematic on-target changes), the way the immune system reacts to CRISPR-Cas tools, and how well different delivery and administration methods work.
Over the past couple of years, there has been encouraging news: Victoria Gray, Jimi Olaghere, and others seem to be functionally cured of sickle cell disease or beta thalassemia, and the edited cells have taken up residence in the bone marrow, indicating the potential for a long-lasting cure. Trials for cancer immunotherapies are at early stages, but the safety and tolerability of the treatments looks promising for moving forward with more newer versions of editing technology, off-the-shelf products, for moving towards new cancer targets, and even developing new cell types for immunotherapy. The initial safety results for treating LCA10 and chronic UTI are positive and we hope to get more efficacy data over the next year. The preliminary results from the hATTR trial are particularly encouraging for this disease and a wide range of diseases with liver pathology, including the new HAE trial. New trials started this year also widen the scope of CRISPR applications to include more common diseases: HIV/AIDS and type 1 diabetes.
All of the treatments are relatively new. Positive results still require long-term follow-up to see if the treatment remains effective, whether patients suffer ill effects from unwanted edits, and whether there are immune reactions in patients with virally delivered Cas proteins.
MORE CRISPR FIRSTS TO LOOK OUT FOR
While the current CRISPR clinical trials are exciting, they focus on the basic capabilities of CRISPR-Cas enzymes and offer only a glimpse of their therapeutic potential. Future milestones will help us learn more about CRISPR’s ability to treat or even prevent diseases:
- A CRISPR treatment that involves inserting DNA to repair or replace a harmful DNA sequence, in essence “pasting” in new material, is still coming. The Graphite Bio sickle cell disease trial will be the first to attempt to directly correct a mutation back to the healthy variant.
- A CRISPR therapy that edits multiple genes at the same time, also known as multiplex editing. Researchers have achieved impressive feats in isolated cells and animal models, and multiplex editing for cancer immunotherapy is currently being developed.
- A treatment that uses base editing. Base editing uses CRISPR components to directly change single DNA letters without making double-stranded breaks in the DNA. For diseases caused by single-letter changes to DNA, base editors may be a safer editing option than conventional CRISPR. Several base-editing treatments are being developed for clinical trials, including for sickle cell disease.
- A treatment that uses prime editing. Prime editing, like base editing, uses CRISPR components to make changes to DNA without making double-stranded breaks. Prime editors can potentially change both single bases and longer stretches of DNA, but has not yet been applied therapeutically.
- A trial where CRISPR tools are used to turn genes on and off without changing the DNA sequence. These strategies, known as CRISPR activation and CRISPR inhibition, don’t require making breaks in a patient’s DNA, so they might be a safer option than conventional CRISPR. CRISPRi and CRISPRa may be reversible — another potential advantage for some applications.
MORE INFO ON CRISPR CLINICAL TRIALS
If you or a loved one are interested in participating in a clinical trial, learn more about how US-based clinical trials work and where to find them on our Patients & Families page. Discuss all important medical decisions with your doctor. Keep in mind that clinical trials are the first tests of new medical treatments, so they are inherently risky and never guaranteed to be successful.
Thanks to Megan Hochstrasser, Fyodor Urnov, Matthew Kan, Bruce Conklin, Alex Marson, Melanie Ott, and John Flannery for insight and editing, and to Dave Ousterout, CSO of Locus Biosciences, for information on their phage therapy program for chronic UTI.