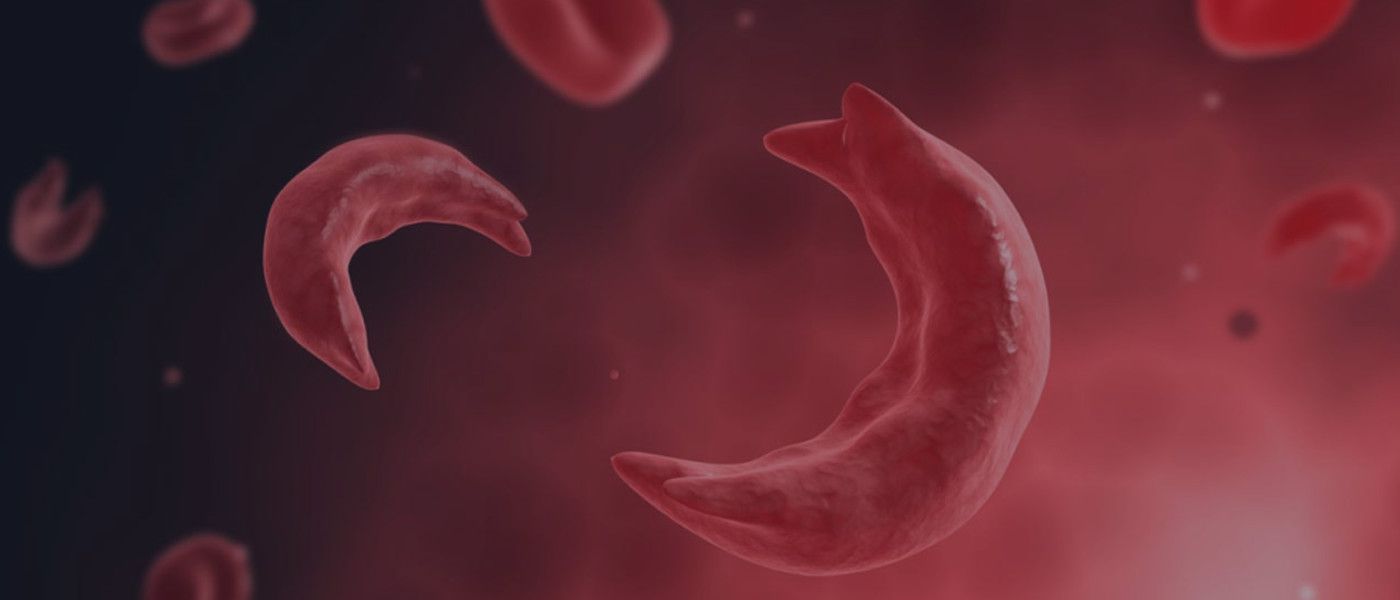
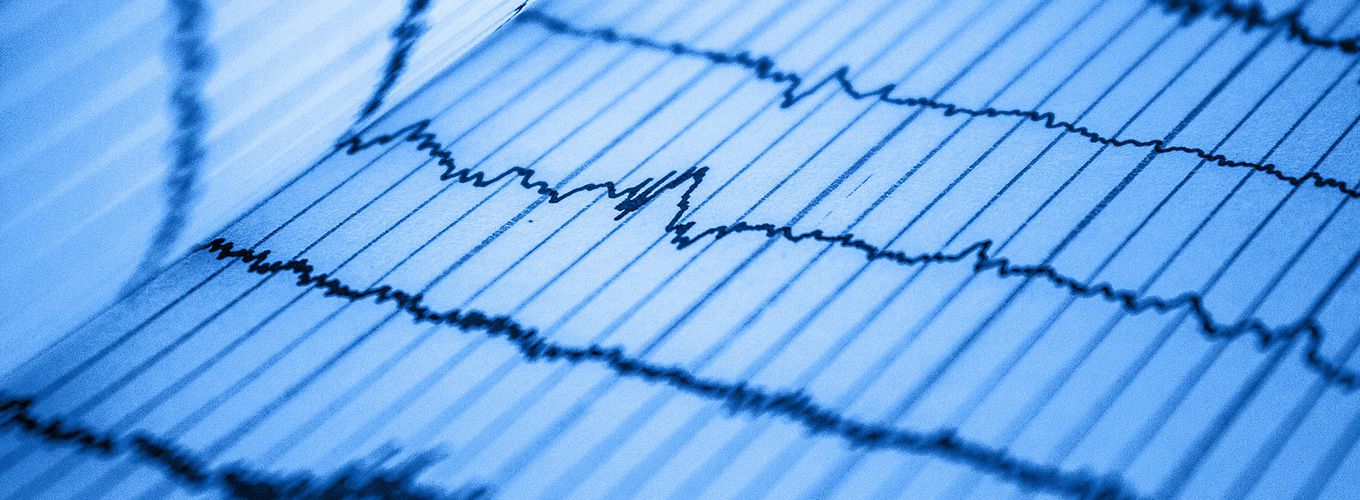
*See our 2023 clinical trials review here.
With the recent buzz about CRISPR clinical trials, we thought it was time for a comprehensive status update! It can be hard to keep track of all the different trials, especially since many of the same diseases pop up in the news as researchers test different treatment approaches. In this post, I’ll introduce you to the basics of clinical trials and then map out the current CRISPR-based trials from disease background to progress updates and what we really hope to learn from these trials.
Clinical trial basics
In the United States, the Food and Drug Administration (FDA) tests new disease treatments for safety and efficacy through clinical trials on patient volunteers. Early trials look at safety and side effects, while later trials test efficacy and compare new therapies with standard treatments.
The current trials using CRISPR therapies are all in the early stages. That means that even if they’re successful, they’re probably still a few years away from FDA approval.
Clinical trials are underway in three treatment areas: cancers, blood disorders, and eye disease. All current CRISPR clinical trials are intended to edit the specific tissues without affecting sperm or eggs, meaning no DNA changes can be passed onto future generations.
CANCERS
Disease background
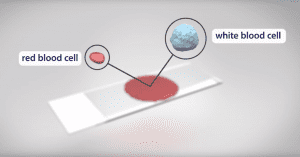
Cancer refers to a group of diseases involving uncontrolled cell growth. Right now, CRISPR-based therapies are mainly aimed at treating blood cancers like leukemia and lymphoma.
Treatment strategy
T cells, better known as white blood cells, are covered in receptors that recognize other cells as safe or threatening. They patrol the body, killing foreign or dangerous cells. In CAR-T immunotherapy, researchers genetically engineer a patient’s T cells to have a receptor that recognizes the patient’s cancer cells, telling the T cells to attack.
The immune system is highly regulated to avoid attacking healthy cells. Some T cell receptors work as “checkpoints” that determine whether an immune response occurs. When a T cell PD-1 receptor comes in contact with a molecule called PD-L1 on another cell, it communicates that it is a “safe” cell and the T cell leaves it alone.
Cancer cells are clever and often cloak themselves in these molecular safety signals, tricking the patrolling T cells into ignoring them. Researchers are using CRISPR to edit the PD-1 gene in T cells to stop them from making functional PD-1 receptors so they can’t be fooled by cancer cells. This immunotherapy approach is known as checkpoint inhibition, and it is used in conjunction with CAR-T engineering to give T cells the greatest possible chance of eliminating cancer.
In these treatments, researchers harvest T cells from a patient’s blood, and engineer them in a lab. This is a kind of ex vivo genome editing, because the genome editing occurs outside of the patient’s body. Ex vivo editing guarantees that genome editing tools only come in contact with the right target cells.
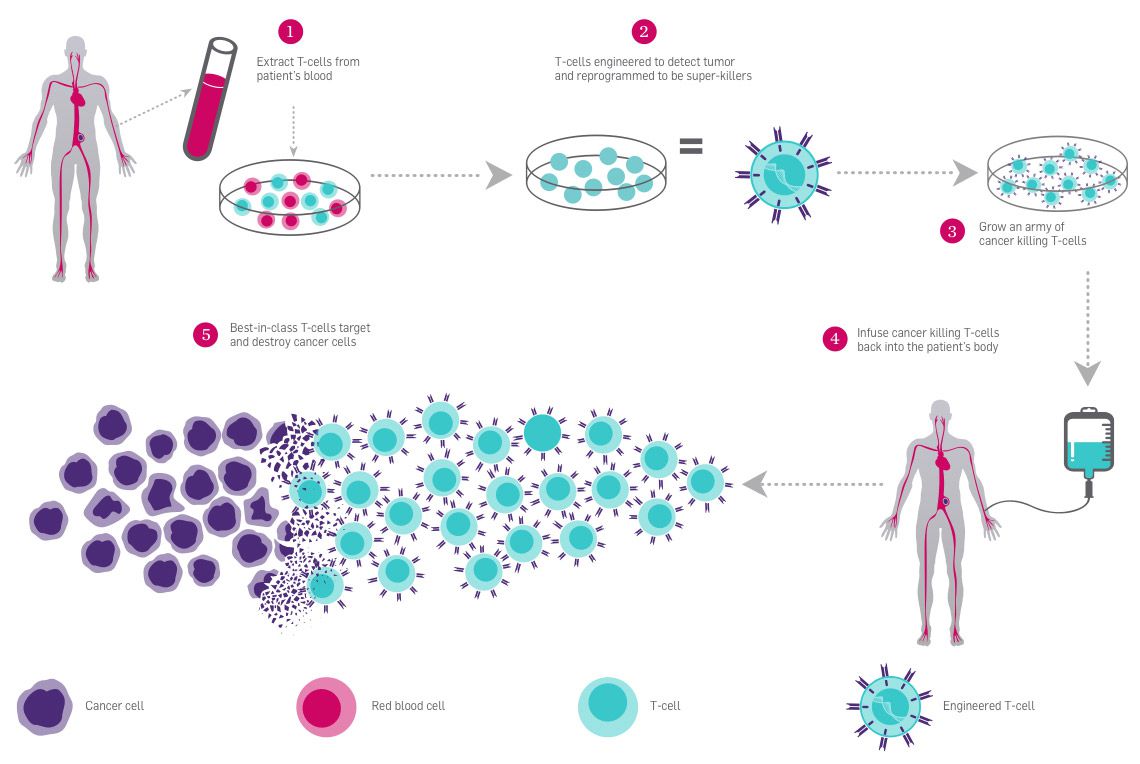
One of the big challenges for genetic therapies is delivery–that is, getting the medicine where it needs to be, and only where it needs to be. Blood cancers are some of the first targets for CRISPR therapies because the delivery is the most straightforward. Modified T cells can be delivered to the blood by IV, like getting a blood transfusion.
Current clinical trials
The first CRISPR-based therapy trial in the US combines CAR-T and PD-1 immunotherapy approaches. This study is currently underway at the University of Pennsylvania, in conjunction with the Parker Institute. Patient volunteers have late-stage cancers and few other treatment options. At least two patients have been treated, but we don’t know the outcomes yet.
Clinical trials testing CRISPR immunotherapies to treat cancer may have begun as early as 2015 in China. Seven active or recruiting trials in China are listed on the US clinical trial database. While China is investing heavily in CRISPR, there are major concerns that clinicians are moving forward without proper clinical trial infrastructure, oversight, safety precautions, informed consent of patients, or research rigor. China has had repeated issues with fraudulent clinical trial data. Little data from active or completed studies has been released.
What to watch for
The FDA has already approved CAR-T therapies and PD-1 pathway inhibitors that don’t use genome editing. This is reason to be optimistic: the proof-of-principle work for these therapies has already been done.
That said, there’s still lots to look out for. While antibody treatments are available to disable PD-1, the efficacy of editing DNA to disable the PD-1 gene hasn’t been tested. In what percentage of cells will it work? Will genetic checkpoint inhibition work as well as current checkpoint-blocking treatments?
These trials should also provide insight into broader questions about CRISPR-based editing: what sorts of changes will happen at the site of the DNA break that Cas9 creates? How often will we see bigger DNA rearrangements than expected? Will there be significant “off-target” changes? Will these risks prove to be more or less important than the benefits?
BLOOD DISORDERS
Disease background
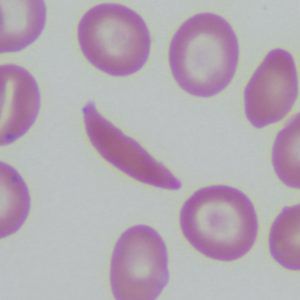
Red blood cells use hemoglobin to pick up oxygen in the lungs and carry it to all the tissues of the body. Mutations in a gene that makes up part of the hemoglobin molecule cause two different genetic disorders: beta thalassemia and sickle cell disease (SCD).
In beta thalassemia, patients do not make enough hemoglobin. This leads to anemia and fatigue. In more severe cases, patients have organ damage, especially to the liver, bones, and heart. In sickle cell disease (SCD), red blood cells become misshapen. Their crescent or sickle shape makes them block blood vessels, slowing or stopping blood flow. This causes sudden, severe pain. Complications include chronic pain, organ damage, strokes, and anemia. Both diseases can be fatal.
There are some treatments available, but these patients still often suffer severe symptoms and complications from their diseases. Patients need frequent blood transfusions. Bone marrow transplant can be curative; however, this is a risky procedure and can only be done when a healthy, matching donor can be found. This is not an option for most beta thalassemia or SCD patients.
Treatment strategy
Current CRISPR clinical trials for blood disorders use a clever workaround: instead of fixing the disease-causing mutations, they aim to increase levels of fetal hemoglobin. This is a form of hemoglobin that fetuses make in the womb, but children and adults don’t usually make. We don’t know why humans switch from one form of hemoglobin to the other, but fetal hemoglobin can take the place of defective adult hemoglobin in red blood cells.
The same genetic treatment targeting fetal hemoglobin can be used to treat beta thalassemia and SCD. The first step of this treatment is to harvest a patient’s blood stem cells from their blood. Next, scientists edit the genomes of these cells to turn on fetal hemoglobin. Then, chemotherapy removes the patient’s remaining defective stem cells, and billions of genome-edited stem cells are put back into the patient’s body. Ideally these cells will create a new blood stem cell population in the bone marrow, which will make red blood cells with fetal hemoglobin.
Gene-edited blood stem cells can be delivered by IV, and ex vivo editing makes it easy to deliver genome editing tools to the target cells.
Trials
In the first use of an ex vivo CRISPR-based therapy to treat a genetic disease, researchers have treated at least one patient with beta thalassemia in Germany. CRISPR Therapeutics and Vertex Pharmaceuticals are running this trial and recruiting patients in Europe and Canada. CRISPR Therapeutics recently revealed that the edited cells seem to be working in their first patient!
In the first use of an ex vivo CRISPR-based therapy to treat a genetic disease in the US, at least one patient with SCD has been treated with the same therapy in Nashville, Tennessee. Early data from this participant look promising; you can follow updates here. The trial is recruiting in the US, Europe, and Canada.
What to watch for
Right now, we don’t know how effective these treatments will be. Key results to look out for include: what percentage of cells are successfully edited? How well do the edited stem cells make a new home in the bone marrow? Will the edited cells make enough fetal hemoglobin to manage a patient’s disease? Over time, will blood stem cells with the edit persist or be replaced by cells with the disease-causing mutation(s)?
Right now, there is a burst of research in blood disorders. Other pharmaceutical and biotech companies are working on both standard and genetic therapies. We don’t know yet which approaches will be most effective, but patients are sure to benefit from renewing interest in these diseases.
EYE DISEASE
Disease background

Leber Congenital Amaurosis (LCA) is the most common cause of inherited childhood blindness. LCA10 is the most common form of LCA. This genetic disease causes severe vision loss or blindness within the first few months of life.
Photoreceptor cells in the eye convert light into nerve signals that travel to the brain. In LCA10, a mutation in a photoreceptor gene makes a shortened, defective version of a crucial protein, making photoreceptor cells dysfunctional. Patients with LCA10 can receive light to the eye, but dysfunctional photoreceptor cells don’t send messages to the brain. Without communication between the eyes and the brain, patients have vision loss or blindness.
Treatment strategy
The CRISPR treatment for LCA10 makes a change to the patient’s faulty photoreceptor gene so that it once again makes a full-size, functional protein instead of the short, broken version. If enough cells are edited and can make the healthy protein, patients should regain vision.
Researchers will give patient volunteers a single dose of the CRISPR therapy by injecting it directly into the patient’s eye. The injection has a nonpathogenic virus (AAV) carrying the Cas9 protein and its guide RNA. Viruses are commonly used in gene therapy and genome editing because they have a natural ability to get into cells. For the LCA10 treatment, the viral vector is engineered so the therapy is active only in photoreceptor cells.
This approach is distinct from those being tested for cancer and blood disorders because it is an in vivo treatment, where the genome editing occurs inside of the patient’s body. Compared to ex vivo editing, in vivo editing has greater challenges and risks. One of the biggest risks is that viral delivery tools or genome editing parts will provoke dangerous immune reactions in a patient. Another big challenge is making sure that CRISPR enzymes don’t stick around too long, since that would give them a greater chance of cutting in unwanted places in the DNA.
You may be a little squeamish thinking about needles near eyes, but the eye is actually an ideal organ for in vivo editing.
It is relatively small so it only requires a small-volume, single-dose treatment. The eye has less immune reactivity compared to most tissues, making a dangerous immune reaction less likely. Because the eye is relatively contained, the CRISPR components are unlikely to travel to other parts of the body, so there is less risk of unwanted genome editing or immune responses in other tissues.
Trial
The FDA has approved human testing of this LCA10 treatment. This will be the first in vivo CRISPR therapy trial. Editas Medicine is running this trial in collaboration with Allergan, and they are currently recruiting patient volunteers throughout the US.
What to watch for
Researchers will need to follow patients closely to see if cells other than photoreceptor cells are affected, or if patients have immune reactions against the treatment. Other key research questions include: what percentage of cells will get effectively edited? Will it be enough to restore vision?
If the treatment works, this will be the first demonstration of a direct fix for a genetic disease. Traditional gene therapies, like those for “bubble boy disease,” work by adding an extra gene–not fixing the original, faulty gene. Likewise, CRISPR therapies for beta thalassemia and SCD don’t fix the original, disease-causing mutation. Success in this trial would be a major step forward in genetic medicine.
THE BIG PICTURE: WHAT WE HOPE TO LEARN FROM THESE TRIALS
Taken together, these trials should help scientists learn about the types of DNA changes that CRISPR enzymes cause in a variety of different tissue types, using different delivery methods. Are there unwanted changes, like big stretches of DNA being cut out or pieces of chromosomes being rearranged? We can assume that unwanted changes will occur at some level, but will they have a meaningful effect on patients? How big of an issue will these be compared to the severity of the diseases being treated?
What these trials have in common is easy delivery options and a low risk of dangerous immune response to the CRISPR components– these therapies are first because there are convenient solutions to these technical challenges. So what’s next?
More medical CRISPR firsts to look out for
While these trials are exciting, there is precedent for cancer immunotherapies and for treating blood disorders by increasing fetal hemoglobin– these are not fundamentally new treatment approaches that hinge upon using CRISPR. Plus, they rely on making making edits to the DNA that are pretty easy for CRISPR-Cas enzymes– they are not really major tests of what CRISPR technology itself can do. In terms of understanding the further reaches of CRISPR’s potential, we’ll learn a lot from future milestones:
- A CRISPR treatment that involves inserting DNA to repair or replace a faulty sequence– “pasting” in new material is still a major challenge.
- A trial where CRISPR tools are used to turn genes on and off without editing DNA. These strategies, known as CRISPR activation and inhibition, don’t require making breaks in a patient’s DNA, so they might be safer. However, it’s unclear how long their effects will last in humans.
- A CRISPR therapy that repairs multiple genes at once. Many major conditions, like diabetes and heart disease are “polygenic,” meaning multiple genes play a role in their progression. While scientists have achieved impressive feats in isolated cells and animal models, we’re a long way from making multiple changes to the genome in real patients.
- An in vivo CRISPR trial that uses non-viral delivery– current technologies rely on viral delivery, which can trigger immune reactions or unwanted insertions of viral DNA into the genome. The small carrying capacity of these viruses also limits what can be done, since a single CRISPR enzyme gene plus its guide RNA usually take up most of the cargo space. Researchers are developing alternative delivery strategies, from nanoparticles and RNPs to exosomes and beyond.
More info on clinical trials
If you or a loved one are interested in becoming part of a clinical trial, you can learn more about how US clinical trials work here and search current trials here. Always discuss important medical decisions with your doctor. Please keep in mind that clinical trials are the first tests of new medical treatments in humans, so they are inherently risky to patients and never guaranteed to be successful.