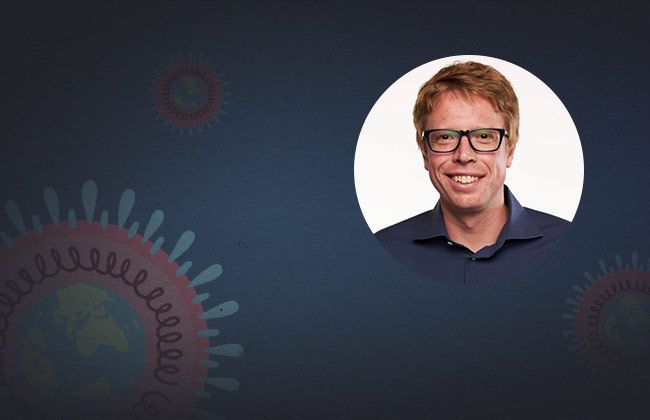
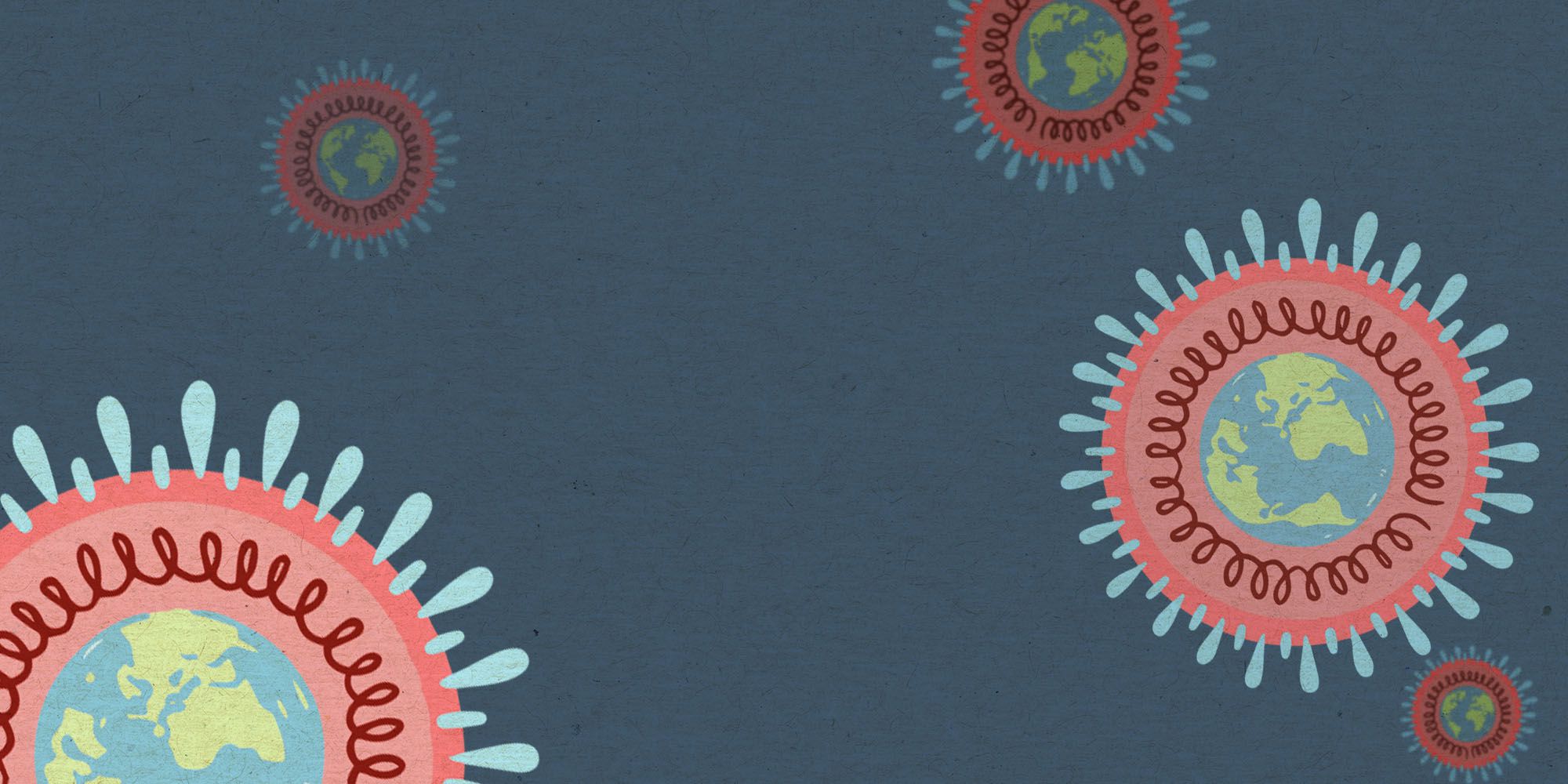
Hiten Madhani is a Professor of Biochemistry and Biophysics at the UCSF School of Medicine, where his lab studies host-pathogen interactions in fungal infections. He is co-leading an IGI-funded research project using yeast to generate weakened versions of SARS-CoV-2, the virus that causes COVID-19, that could then be used to make a live-attenuated vaccine. In September, he talked with IGI science writer Hope Henderson about this project.
What’s the goal of your project?
We’re working towards developing a vaccine for SARS-CoV-2. The goal of the project is to develop tools that will allow us to engineer the virus easily, and use them to produce a weakened form of the virus called a live-attenuated virus that won’t cause disease in people but could be used to safely immunize us against the virus.
A lot of people are working on vaccine efforts. How does your project stand out?
I’m glad that lots of people are working on vaccines! A large fraction of vaccines fail in trials at various stages. So, we need many different shots towards the goal!
While no vaccine is a hundred percent effective, historically, live-attenuated vaccines are the best at preventing transmission, preventing infection, and preventing disease.
Those are actually three different end points: whether somebody gets sick, whether somebody has virus replicating in their nose, and whether they transmit it.

Another thing worth mentioning is that scaling up production of a live-attenuated vaccine is easy because they’ve been used for many, many years. Basically all you have to do is grow a lot of the safe version of the virus in cells. The MMR vaccine that every kid gets is actually three live attenuated-vaccines in one, measles, mumps, rubella, with an extraordinarily high efficacy of 85 to 95%. For parts of the world that don’t have a lot of resources, it’s hard to imagine anything but a live-attenuated vaccine that is cheap and easy enough to produce and store.
Finally, this would actually be the first time CRISPR is used to generate a live-attenuated vaccine.
Is there a possibility that a vaccine you generate will be applicable not just to SARS-CoV-2, but to future coronaviruses?
One of the advantages of live-attenuated vaccines is that because you’re expressing all of the viral proteins, you have the potential for people to make a number of different antibodies that respond to viral proteins, many of which are virtually identical amongst coronaviruses. The AB protein in particular is remarkably similar across these proteins. So, there’s a potential for a live-attenuated vaccine against SARS CoV-2 to protect or give some protection against all of these viruses. If you could generate long-lived immune responses they might be enough to at least prevent severe disease.
The other benefit would be, we can use the viruses we’ve engineered for this vaccine on future viruses. If a new coronavirus emerged with a significantly different Spike protein, for example, we could swap out the proteins very quickly, and make a targeted live-attenuated vaccine for the new coronavirus.
That was a very compelling argument for live attenuated vaccines!
Yeah, it’s hard to argue with it. They’re not easy to make though. Nobody else outside of a couple groups in India who are doing codon optimization are doing it. That’s because it’s harder to do. It’s less predictable. And people are concerned about safety. But again, the measles, mumps, and rubella vaccines are live-attenuated and very safe. You just have to do the appropriate safety studies. And it just takes longer.
Do you see a scenario where there are earlier vaccines that are further along and maybe a couple get approved and have some effect, but a more effective live-attenuated vaccine comes out later?
Certainly that will be the case. I mean, there’s just no doubt about it. Another thing to keep in mind is that vaccine efficacy can be very different in different populations, like children, adults, and the elderly. So those are huge unknowns.
So we might get different vaccines, first, in some places or for some populations, as stop-gap measures.
I hope so!
Do you have any comments about what’s happening in Russia, where a vaccine was approved without phase 3 trials?
I don’t know enough about it to comment very intelligently, besides to say what I hope any scientists would say, which is that it’s important to demonstrate that a vaccine is safe and efficacious before administering it to large populations.
So, you’ve been working with yeast. What do yeast have to do with making live-attenuated viruses?
With any virus, if you want to manipulate its genome, you have to be able to get it into a DNA form. Usually you can do that by using bacteria as a factory to make copies of it. Coronaviruses have long genomes and seem to be toxic to bacteria, so it’s really tricky to work with them that way. A group reported in a preprint in February that they could do it in yeast, which is something I have expertise in. And Raul Andino got in touch with me about collaborating to do this. We’ve found that yeast can propagate a DNA form of the viral genome very well. And, CRISPR engineering works really well in yeast! In fact, it probably works better in yeast than in any other organism. And so we have modified existing CRISPR tools to use on the coronavirus genome in the yeast. We can delete each of the individual genes of the virus and sequence the whole genome. As advertised, it works to make precise deletions or mutations using CRISPR. The step in the process, where we are now, is isolating enough of the genome from yeast. What do you do once you have enough copies of the genome from yeast?
The next goal is to make attenuated virus — that is, complete viral particles that are made safe.
So how do you make a virus starting with DNA? You make an RNA copy in a test tube from that DNA that you’ve purified from yeast, and then you introduce that RNA to mammalian cells. We can get virus RNA replication to happen in the cells, but we have yet to produce the complete viral particles. It’s challenging, so we’re taking several approaches. It’s forcing us to innovate beyond the existing protocols.
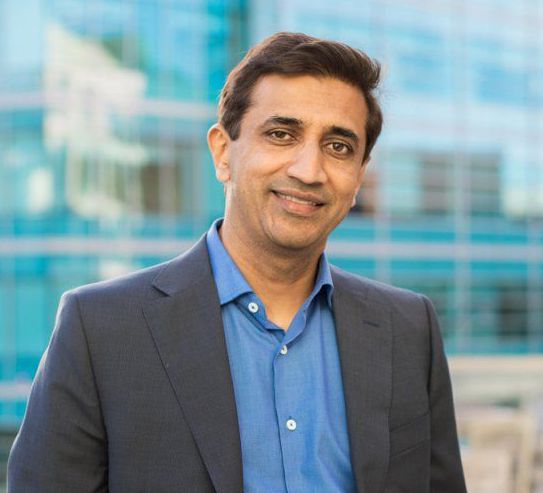
Presuming you’re able to get the viral particles to assemble, what will the next few steps be?
Next, we introduce mutations — single mutations or two at a time — to the viral genome. We’re thinking of 70 or 80 different mutated viruses. The genes we’re targeting are based primarily on the literature with SARS-1. The goal is to make mutations that change the viruses so it’s not dangerous, without making it so the virus can’t survive. After that, we cause disease in research animals. We have a mouse that has the human ACE2 receptor that the coronavirus uses to get into cells. The disease is less severe in rodent models, so we’re also working on making a mouse model that gets a disease that looks closer to the human disease.
What are things like in your lab right now?
My researchers are there, but I’m working from home. I’m very impressed by the resilience of people in my lab. They can effectively work together under these circumstances even though they each have different hours, have different constraints on their schedule, and some have children at home that they’re homeschooling. But I mean — last Wednesday, with the smoke from the wildfires. You look out the window and, Oh my God.
That was very unsettling.
It reminded me of when I was a kid and Mount St. Helens erupted. It became the dead of night during the day, and that area was blacked out for days. I grew up just outside of Seattle, so it was a local story, and some ash eruptions that dropped on Seattle. I remember coming downstairs and everyone being transfixed on the television.
Have you developed any new quarantine hobbies?
My wife, Suzanne Noble, who’s also a professor at UCSF, has been busy with a grant. She had been doing most of the cooking and she told me to take over. I bought a new, high-end, stainless steel, infrared barbecue. I’ve been barbecuing dinner every night since then!
Do you have any specialties on the grill?
You wanna hear my rotation? Salmon with dill, catfish with paprika and garlic, burgers, sometimes Impossible Burgers, and chicken tikka masala.
This work is funded by the Laboratory for Genomics Research (LGR), a collaboration between UC Berkeley/UCSF (IGI) and GlaxoSmithKline.